Quantum optics
Light particles interacting with matter
Quantum optics describes how individual quanta of light, the photons, interact with atoms, molecules and larger pieces of matter. We focus on studies of light interacting with nanoscale systems, in both experiment and theory. Our motivation for this is to create and study hybridized quantum states between electrons and photons. We also aim for example to increase the coherence of these states and their interaction strength to build electro-optical systems, devices and sensors.
Research areas:
Microwave photonics
Microwave photons are one of the main interaction media in solid-state quantum devices such as superconducting and semiconducting qubits. We study experimentally and theoretically microwave photons interacting with electrons in semiconductor nanostructures in so-called circuit quantum electrodynamics (cQED) setup. For the electronic part of the system, we focus on using quantum dots that enable to build photodetectors in the microwave domain and strong coherent coupling between electric charge and microwave photons in the electrical circuits.
Many-Body Physics of Finite Quantum Systems
Research in this area sets focus on the theory of quantum-optically confined systems of ultra-cold atoms and molecules and related nanostructures. We work on novel quantum systems at the crossover between the few- and many-body limit, studying precursors of phase transitions and hitherto unknown states of quantum matter, such as self-bound bosonic quantum droplets or dipolar supersolidity. We focus on fundamental theoretical questions while working closely in contact with experiment.
Quantum Light Spectroscopy
We develop detectors and spectroscopy tapping into the quantum properties of the entangled photons and single photon detectors with high quantum efficiency. These techniques hold the promise to provide access to nontrivial quantum dynamics with superior sensitivity as compared to the common spectroscopies made with classical light fields.
Key publications:
- Haldar, S. et al. Energetics of Microwaves Probed by Double Quantum Dot Absorption. Phys. Rev. Lett. 130, 087003
- Khan, W. et al. Efficient and continuous microwave photoconversion in hybrid cavity-semiconductor nanowire double quantum dot diodes. Nature Communications. 12, 1, 5130.
- Sand Kalaee, A. A. et al, Violating the thermodynamic uncertainty relation in the three-level maser. Phys. Rev. E 104, L012103
- Bergenfeldt, C. et al. Hybrid Microwave-Cavity Heat Engine. Physical Review Letters. 112, 7, 076803.
Contact persons:
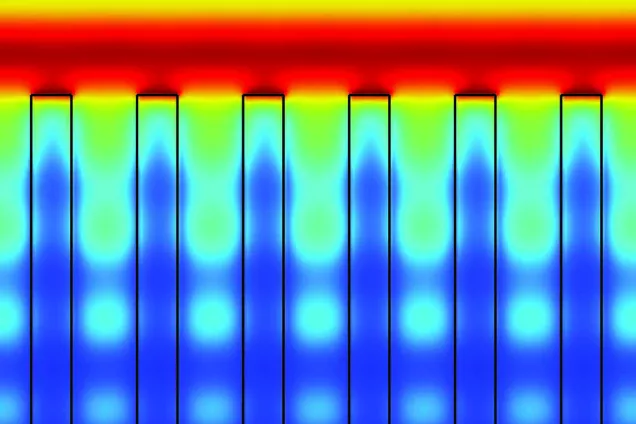
Nano-Tandem
Research to develop tandem architecture for high-efficiency photovoltaics (H2020, 2014-2019)
QPHOTON
Microwave Quantum Photonics for Quantum Technology and Fundamental Physics (QPHOTON)
European Research Council Consolidator Grant